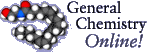
 Home Common Compounds Exam Guide FAQ Features Glossary Construction Kits Companion Notes Just Ask Antoine! Simulations Slide Index Toolbox Tutorial Index Companion Notes
Introduction Measurement Matter Atoms & ions Compounds Chemical change The mole Gases Energy & change Electrons in atoms The periodic table
|  | 

Learning objectives
- Relate wavelength
, frequency , and velocity of waves.
- Explain how electromagnetic radiation
is produced by an oscillating charge.
- Explain how electromagnetic radiation carries energy from a transmitter to a receiver.
- Describe the collapsing atom paradox.
- List wave behaviors, and distinguish them from particle behaviors.
- Cite experimental evidence that implies that electromagnetic radiation can display both wave and particle behaviors.
- Cite experimental evidence that implies that electrons display both wave and particle behaviors.
- Connect particle and wave properties of matter using de Broglie's hypothesis.
- Explain what a standing wave is.
- Compare a wave on a wire, a particle on a wire, and an electron on a wire.
- Show how de Broglie's hypothesis implies the existence of quantized energy states for standing electron waves.
- Show how quantum numbers arise for standing electron waves.
- State Heisenberg's uncertainty principle, and explain why it resolves the collapsing atom paradox.
Lecture outlineElectrons behave like particles in some experiments, and like waves in others.
The electron's 'wave/particle duality' has no real analogy in the
everyday world. The quantum theory that describes
the behavior of electrons is a cornerstone in modern chemistry.
Quantum theory can be used to explain why atoms are stable, why things have the color they do, why the periodic table has the structure it does, why chemical bonds form, and why different elements combine in different ratios with each other.
Light and electrons both behave quantum mechanically. To understand the experimental basis for the quantum theory, we have to begin our discussion with light.
Waves
Is light a stream of particles or a wave?
- Thomas Young, 1801
- pass light through two tiny adjacent slits
- if light were particles:
- target would be brightest where light passing through the slits overlapped
- target would darken steadily moving away from the overlap region
- this was not observed!
- a pattern of light and dark stripes was observed instead
- Young explained the stripes as a
combination of diffraction and interference
- these interference fringes are a sure sign of wave behavior
- width of interference bands suggested the wavelength of visible light was less than a millionth of a meter!
- If light is a wave, what is oscillating?
- light can move through a vacuum, so it isn't an oscillation of atoms as sound and water waves are.
Force Fields
- force field: a region where forces act on an object; strength of forces vary with position
- gravitational fields
- larger mass at center of field = stronger forces
- larger distance from center of field = weaker forces
- electric fields
- opposite charges attract each other, but like charges repel each other
- larger charge at center of field = stronger forces
- larger distance from center of field = weaker forces
- magnetic fields
- can be produced by moving charges (electromagnets)
- a moving magnetic field can produce an electric field (electric generator)
Electromagnetic radiation
- James Clerk Maxwell ca. 1855
- changes in electric and magnetic fields are always coupled: electromagnetism
- making e/m waves with a vibrating charge
- both electric and magnetic fields oscillate
- oscillations are at right angles
- electric oscillation produces magnetic oscillation, which produces another electric oscillation,
and on and on
- vibrating charge creates a ripple in the electromagnetic field
- The speed of electromagnetic radiation was computed to be around 3×108 m/s
- The same speed had been determined experimentally for light!
- hypothesis: light is a form of electromagnetic radiation (Maxwell, 1862)
The electromagnetic spectrum
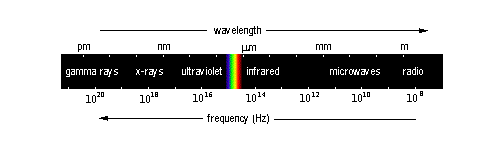
Major regions of the electromagnetic spectrum. The rainbow band represents the visible region. Know the names of the regions and their order with increasing wavelength.
region of spectrum |
wavelengths |
typical source |
radio |
more than 30 cm |
radio, television |
microwave |
3 mm to 30 cm |
radar, microwave oven |
infrared |
750 nm to 3 mm |
hot objects |
visible |
400 nm to 750 nm |
very hot objects |
ultraviolet |
20-400 nm |
sun; black lights |
x-rays |
3 pm to 20 nm |
cathode ray tubes |
gamma rays |
less than 3 pm |
Energy of electromagnetic radiation
- radiation carries energy through space
- work is done on charges in the e/m field
- transmitter loses energy; reciever gains energy
- for classical waves:

- higher amplitude means higher energy per peak
- amplitude squared determines the intensity or brightness of light
- therefore, brighter light should carry more energy per peak than dimmer light
- an experiment to measure the energy carried by an electromagnetic wave
- photoelectric effect: shining light on alkali metals knocks electrons out of metal
- strategy: measure kinetic energy of ejected electrons; then measure light energy per ejected electron.
- surprise:
- brightness has NO EFFECT on the kinetic energy per ejected electron
- brighter light ejects MORE electrons.
- surprise #2:
- red light can't eject any electrons, but blue light can!
- below a threshold frequency (
0), there are no ejected electrons!
0 is a property of the metal being used
- Albert Einstein's interpretion of the photoelectric effect (Nobel Prize, 1921)
- maybe light is like a stream of massless particles (call them photons)
- brighter light has more photons, but bluer light has higher energy photons
- frequency-to-energy conversion factor is h (Planck's constant, 6.626×10-34 J/Hz)
|
Planck used the hypothesis that E = h to explain the spectrum of light emitted by hot bodies. |  |
The collapsing atom paradox
- what's the electron doing in an atom?
- electrons within the atom can't be stationary:
- positively charged nucleus will attract the negatively charged electron
- electron will accelerate towards the nucleus
- if electrons within the atom move,
- moving charges emit electromagnetic radiation
- emission will cause electrons to lose energy and spiral into the nucleus
- the atom will collapse!
- why don't atoms collapse?
- classical physics has no answer!
- key: electrons have wave/particle duality
Electrons as Waves
- the de Broglie hypothesis (Nobel Prize, 1929)
- connect wave and particle nature of matter using a relationship that applies to photons:
= h/p
where p is the momentum of the particle (p = mass times velocity).
- examples
Compute the de Broglie wavelength of a tennis ball (m = 0.1 kg) and an electron (m = 9.1 x 10-31 kg), if both are moving at 1000 m/s.
- experimental evidence of electron wave/particle duality
- electron diffraction
- C. J. Davisson and G. P. Thomson observed interference fringes when electron beams hit crystal surfaces and thin metal films (Nobel Prize, 1937)
 |
Electron diffraction pattern collected from
crystalline silicon Semiconductor Surface Physics Group Queens University |
- electrons passing one at a time through a double slit. Each spot shows an electron impact on the detector.
 | 100 electrons |
 | 3000 electrons |
 | 70000 electrons
interference fringes!!! |
- applications
- LEED surface analysis
- electron microscopy
Bound electrons have quantized energies
- model I: bead on a wire
- kinetic energy of bead can have any value, because velocity can have any value
- bead can be stationary
- bead is equally likely to be found anywhere on the wire
- exact position and velocity of the bead can be known simultaneously
- model II: wave on a wire
- there must be a whole number of peaks and troughs on the wire:
n ( /2) = L, where:
- n is an integer (1, 2, 3, ... )
-
is the wavelength
- L is the length of the wire
- standing waves have quantized wavelengths
- model III: electron on a wire
- unite wave and bead models using the de Broglie relation:
particle kinetic energy: |
 |
de Broglie wave/particle relation: |
|
standing wave allowed wavelengths: |
|
- The fact that E depends on an integer n means that only certain energy states are allowed
- the integer n labels each state; n is a quantum number
- Notice that n can't be zero, so the lowest energy state is not zero: the electron is never at rest!
- peaks and troughs correspond to buildup of negative charge- electron is not equally likely to be found anywhere on the wire.
Summary
- electrons behave like waves
- bound waves have restricted wavelengths
- therefore, electrons bound in atoms and molecules have restricted energies
The uncertainty principle
- Quantum theory puts a limit on the precision of measurements
- Werner Heisenberg's uncertainty principle (Nobel Prize, 1932)
- The uncertainty principle says that the act of measurement changes what you're trying to measure
- You can bounce a photon off a brick wall, and the wall doesn't change much...
- ...but bouncing a photon off an electron to measure its position will change its momentum
- Why atoms don't collapse
- The uncertainty in position is also the smallest space a particle can possibly be restricted to
- The position of an electron can't possible be known to better than ± 4 × 10-13 m by the uncertainty principle
-
so an electron can't be confined within the nucleus
|